www.magazine-industry-usa.com
30
'24
Written on Modified on
ADVANCING SOLAR PANEL EFFICIENCY – INNOVATIONS, CHALLENGES AND THE WAY FORWARD
Milton D’Silva presents an overview of the contemporary Solar PV ecosystem and the challenges, even as innovations continue to propel it forward.
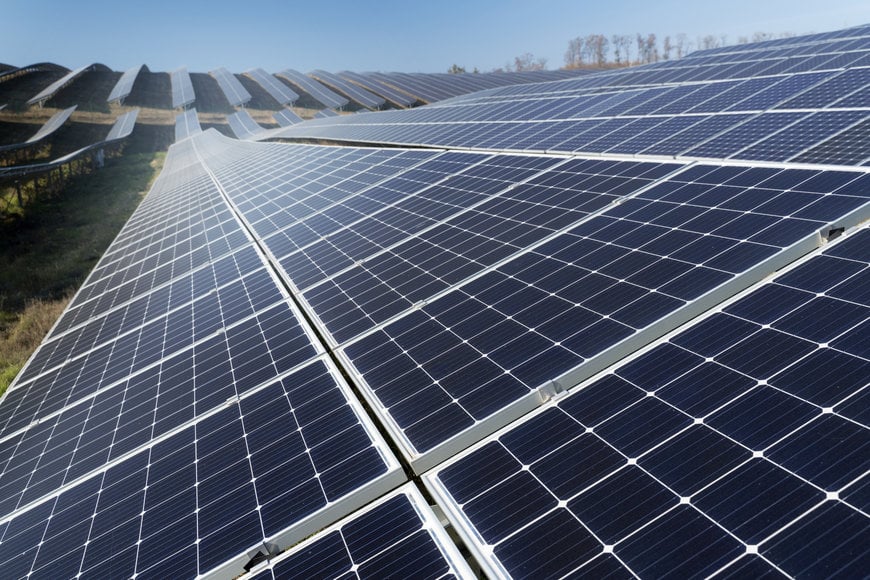
A typical commercial installation of solar PV panels. Image by Freepik
The extreme weather-related events of recent years have amply demonstrated the degradation of Planet Earth by human activities, with a climate crisis looming on the horizon. The year 2023 is said to be the warmest in the recent history of the world, with March 2024 as one of the warmest months. Fossil fuels, which provide almost 80% of the world’s energy needs today, are largely responsible for this climate change owing to their greenhouse gas emissions. The emerging consensus, as the world is slowly contemplating a move towards clean energy, is to achieve a 50% reduction in carbon emissions by 2030 and reach net-zero by 2050. Governments across the world are now investing in clean energy sources, and solar power generation has emerged as one of the cleanest alternatives.
The use of solar energy is not a recent phenomenon – it is as old as mankind. Apart from providing daylight, the sun has always been a source of heat energy that has powered the earth. It is responsible for the water cycle that is so critical to sustain life, as it is responsible for the weather patterns and ocean currents that make the climate changes on earth. However, these are all naturally occurring phenomena without human intervention. What is of recent origin is the use of solar energy to generate electricity. There are two methods for converting solar energy into electricity – directly using photovoltaic (PV) cells or indirectly using concentrated solar-thermal power (CSP). This article concerns the former, and examines at length the evolution of the solar PV cell over the last six decades and the rapid strides made in recent years.
According to Wikipedia, it was French physicist Edmond Becquerel who built the world's first experimental photovoltaic (PV) cell in 1839 in his father's laboratory – he was just 19 then. Over 100 years later – the period marked by efforts of various researchers at demonstrating the practical photoelectric effect – the first practical photovoltaic cell was publicly demonstrated at Bell Laboratories in 1954 by inventors Calvin Souther Fuller, Daryl Chapin and Gerald Pearson. Interestingly, Albert Einstein won the Nobel Prize in 1921 for his landmark paper published in 1905, which had explained a new quantum theory of light. Einstein’s research contributed significantly to the development of photovoltaic technology in subsequent decades. It is the photoelectric effect that is at the heart of the PV cells that form the solar panels, which convert sunlight into electricity with the help of semiconductors that exhibit the photoelectric effect.
The first practical use of solar PV cells for generating electric power was made in 1958 by NASA for the Vanguard 1 satellite, which was the second satellite launched by the US. It may be noted that in late 1957, the then USSR (now Russia) had launched the first artificial satellite in space. The solar cells used in Vanguard1 were meant as an alternative power source to the battery for operating the transmitter, which expectedly did not last more than a couple of months. However, the solar-powered transmitter continued to work for almost six years. Incidentally, this particular satellite is still orbiting the earth, the oldest man-made object in space, and is being tracked optically. With the successful use of solar PV cells in the Vanguard1 satellite, these became the primary power source for all earth-orbiting satellites. The cost of these early solar PV cells was very high – around $100 per watt or more – but they provided the best power-to-weight ratio, which for space applications was a great advantage.
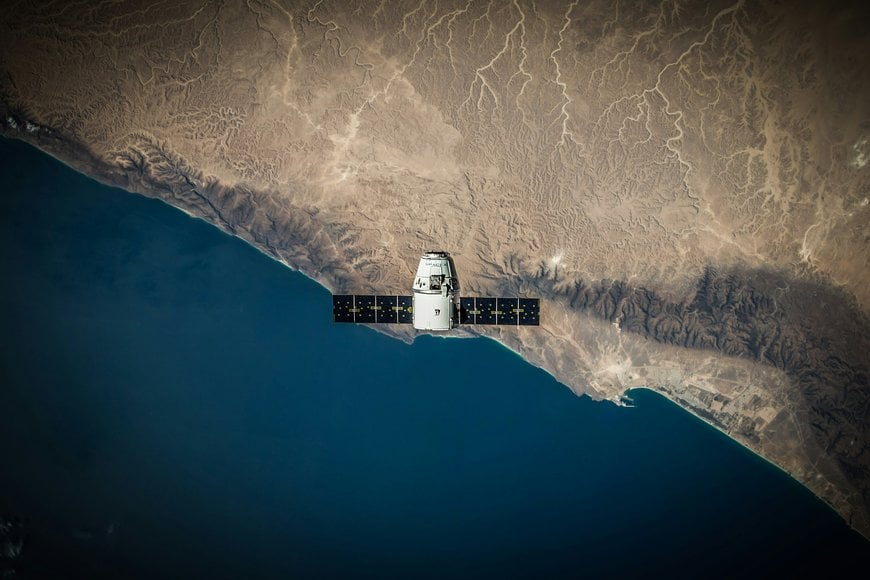
Illustration of a solar panel deployed on a satellite in space. Photo by SpaceX on Unsplash
The impetus for growth of solar energy
As researchers continued to experiment with different materials and semiconductors for improving the efficiency of solar PV cells for aerospace applications, the terrestrial solar panels were not really high on the agenda, but nevertheless benefitted from the research in terms of efficiency improvement. Ironically, the much-maligned oil industry – more appropriately, one of the leading American oil companies, Exxon – was instrumental in the growth of the solar PV cells industry in the early years, but more of that later.
How exactly do solar PV cells work? Solar PV cells work by converting sunlight directly into electricity. Each PV cell is made of semiconductor materials, like silicon, which have special properties. In actual operation, the photons in the sunlight are absorbed by the semiconductor which causes the movement of the electrons, causing them to flow and create an electric current. This flow of electrons generates direct current (DC) electricity. The PV cells are connected together in a panel, and multiple panels are connected to form a solar array. The electricity produced by the solar array can then be used to power homes, buildings, or other electrical devices.
If the oil shocks of the late 1960s and early 1970s made the world aware of the potential of oil as a weapon, even before that, Exxon was the first oil company to understand the finite nature of this precious fossil source of energy and had begun to hedge its bet on other possible revenue streams. As the solar PV cells helped satellites get a reliable alternative to batteries, the idea caught up with an executive at Exxon as a solution to the batteries powering navigational aids on remote oil platforms. Typically, the batteries did not last long, were expensive to replace, and worse, were often discarded at sea, adding to the pollution. The work that Exxon started then to develop PV cells of its own, later led to the formation of its subsidiary, Solar Power Corporation, in 1973. Thanks to its huge revenues, Exxon could spend a large amount of resources for developing solar PV cells and panels, to help them spread beyond the use in satellites. Yet, in another twist of fate, the slow progress of solar power and its failure to capture popular imagination resulted in Exxon shutting down SPC in the mid-1980s, not willing to fund further research and wait for the dividends to follow. But the biggest blow the Exxon initiative struck for solar PV cells was to drive down the cost of solar power from $100 per watt to $20 a watt and paved the way for further research and reduction.
In the meanwhile, other initiatives followed. In 1977, the US Department of Energy launched the Solar Energy Research Institute (later renamed National Renewable Energy Laboratory) dedicated to research and development of solar energy. Governments and research institutions in other countries worldwide began also funding solar research and development. Efficiency improvements and cost reductions followed.
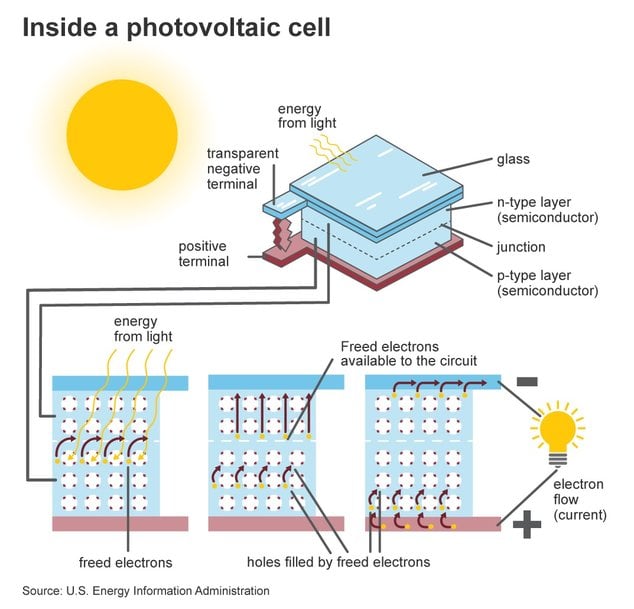
The schematic of a typical solar PV cell. Source: US Energy Information Department
Solar cell efficiency
According to the website of the US Government’s Office of Energy Efficiency & Renewable Energy, the amount of sunlight that strikes the earth's surface in an hour and a half is enough to handle the entire world's energy consumption for a full year. In reality though, it is not possible to convert this entire sunlight into electricity or useful thermal energy, and that is where the efficiency of the solar PV cell enters the equation, which is the amount of usable electrical power a solar cell produces from the sunlight shining on it. This efficiency is expressed as a percentage and represents the ratio of electrical power output from the solar cell to the solar power input from the sun. In simpler terms, it measures how effectively a solar cell can convert sunlight into electricity.
The efficiency of solar PV cells has been improving continuously with every passing year and development of new materials and processes. For example, the first practical solar cell developed by Bell Laboratories in 1954 had an efficiency of just around 6%. The progress in the initial years was very slow and in the decade that followed, efficiencies remained relatively low, typically below 10%. During the 1980s, efficiencies began to increase due to advancements in materials and manufacturing techniques and reached around 15%. The 1990s witnessed further improvements in materials and manufacturing processes that pushed efficiencies up to about 20%.
In the new millennium, efficiencies continued to climb steadily. By the end of the decade, efficiencies of around 25% were achieved in laboratory settings for some high-performance solar cells. Post-2010s, continued research and development led to incremental improvements, with efficiencies surpassing 30% in laboratory settings for certain types of solar cells. Technological advancements, including the development of new materials, improved cell designs, and enhanced manufacturing processes, have led to efficiencies exceeding 40% in laboratory settings for some cutting-edge solar cell technologies in the present time. But it may be noted that while laboratory efficiencies have continued to rise, the efficiencies of commercial solar panels available on the market today are typically only about 25% maximum.
There are several factors that impact the efficiency of solar PV cells and modules. These broadly include the intensity of the sunlight, cloud cover, the temperature of the solar panel, wind speed and altitude at which these panels are placed. Even the dust that settles on the solar panels impact their efficiency and it is recommended to clean the panels at least once in a year. Higher-efficiency solar cells can produce more electricity for a given amount of sunlight, making them more cost-effective and practical for various applications, including residential and commercial solar power systems. Improving solar cell efficiency is an ongoing goal in solar energy research and development.
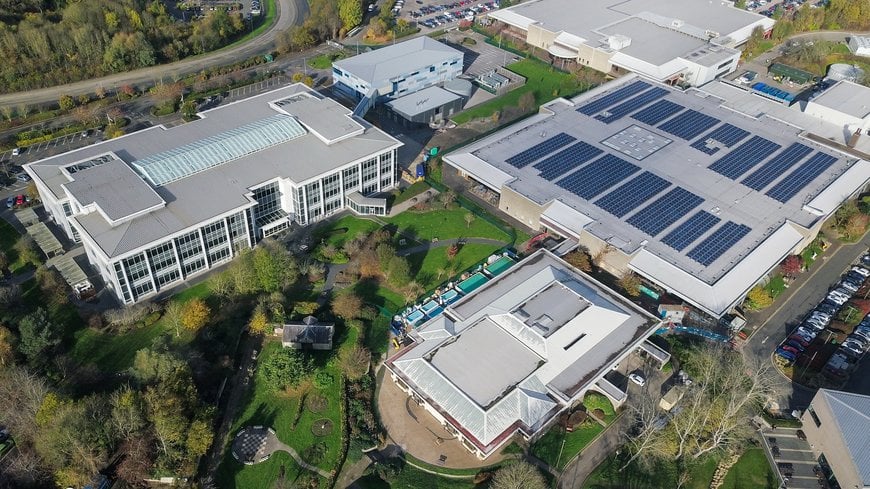
Aerial shot of industrial buildings in Bristol, UK. Photo by wirestock on Freepik
Types of solar PV cells
Solar photovoltaic (PV) cells come in several types, each with its own advantages and applications. Some of the common types of solar PV cells are described below:
Monocrystalline Silicon Solar Cells: These cells are made from a single crystal structure, typically sliced from cylindrical ingots. These were the first commercially available solar PV cells. They are highly efficient and space-saving, making them popular for residential and commercial installations. On the other hand, these cells are expensive and their manufacturing process is slow and labour-intensive.
Polycrystalline Silicon Solar Cells: These cells are made from silicon crystals that are melted together, then sawn and packaged similar to monocrystalline cells. As the name suggests, instead of a single uniform crystal structure, these cells contain many small grains of crystals. They are less expensive to produce than monocrystalline cells but are slightly less efficient. They have a bluish hue due to multiple crystals.
Thin-Film Solar Cells: Thin-film solar cells are made by depositing thin layers of photovoltaic material onto a substrate such as glass, metal, or plastic. Types of thin-film solar cells include:
The extreme weather-related events of recent years have amply demonstrated the degradation of Planet Earth by human activities, with a climate crisis looming on the horizon. The year 2023 is said to be the warmest in the recent history of the world, with March 2024 as one of the warmest months. Fossil fuels, which provide almost 80% of the world’s energy needs today, are largely responsible for this climate change owing to their greenhouse gas emissions. The emerging consensus, as the world is slowly contemplating a move towards clean energy, is to achieve a 50% reduction in carbon emissions by 2030 and reach net-zero by 2050. Governments across the world are now investing in clean energy sources, and solar power generation has emerged as one of the cleanest alternatives.
The use of solar energy is not a recent phenomenon – it is as old as mankind. Apart from providing daylight, the sun has always been a source of heat energy that has powered the earth. It is responsible for the water cycle that is so critical to sustain life, as it is responsible for the weather patterns and ocean currents that make the climate changes on earth. However, these are all naturally occurring phenomena without human intervention. What is of recent origin is the use of solar energy to generate electricity. There are two methods for converting solar energy into electricity – directly using photovoltaic (PV) cells or indirectly using concentrated solar-thermal power (CSP). This article concerns the former, and examines at length the evolution of the solar PV cell over the last six decades and the rapid strides made in recent years.
According to Wikipedia, it was French physicist Edmond Becquerel who built the world's first experimental photovoltaic (PV) cell in 1839 in his father's laboratory – he was just 19 then. Over 100 years later – the period marked by efforts of various researchers at demonstrating the practical photoelectric effect – the first practical photovoltaic cell was publicly demonstrated at Bell Laboratories in 1954 by inventors Calvin Souther Fuller, Daryl Chapin and Gerald Pearson. Interestingly, Albert Einstein won the Nobel Prize in 1921 for his landmark paper published in 1905, which had explained a new quantum theory of light. Einstein’s research contributed significantly to the development of photovoltaic technology in subsequent decades. It is the photoelectric effect that is at the heart of the PV cells that form the solar panels, which convert sunlight into electricity with the help of semiconductors that exhibit the photoelectric effect.
The first practical use of solar PV cells for generating electric power was made in 1958 by NASA for the Vanguard 1 satellite, which was the second satellite launched by the US. It may be noted that in late 1957, the then USSR (now Russia) had launched the first artificial satellite in space. The solar cells used in Vanguard1 were meant as an alternative power source to the battery for operating the transmitter, which expectedly did not last more than a couple of months. However, the solar-powered transmitter continued to work for almost six years. Incidentally, this particular satellite is still orbiting the earth, the oldest man-made object in space, and is being tracked optically. With the successful use of solar PV cells in the Vanguard1 satellite, these became the primary power source for all earth-orbiting satellites. The cost of these early solar PV cells was very high – around $100 per watt or more – but they provided the best power-to-weight ratio, which for space applications was a great advantage.
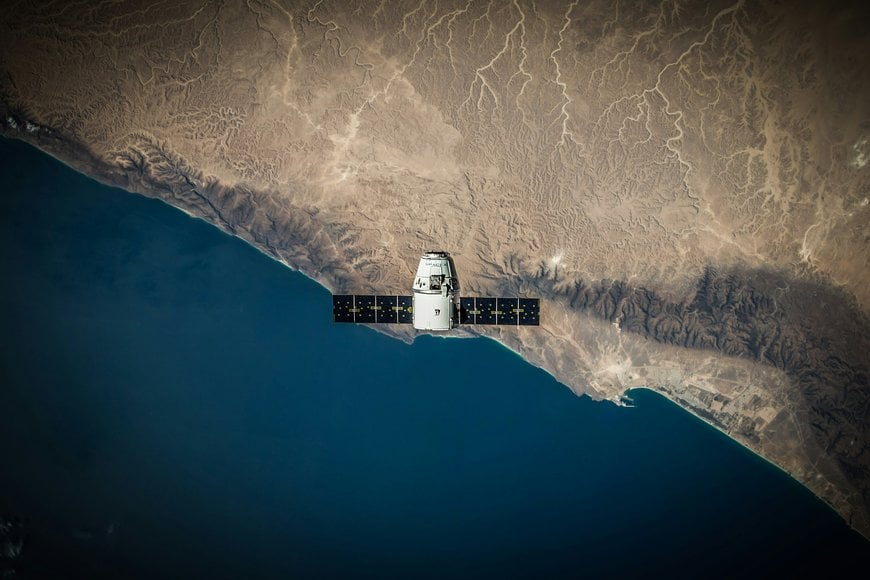
Illustration of a solar panel deployed on a satellite in space. Photo by SpaceX on Unsplash
The impetus for growth of solar energy
As researchers continued to experiment with different materials and semiconductors for improving the efficiency of solar PV cells for aerospace applications, the terrestrial solar panels were not really high on the agenda, but nevertheless benefitted from the research in terms of efficiency improvement. Ironically, the much-maligned oil industry – more appropriately, one of the leading American oil companies, Exxon – was instrumental in the growth of the solar PV cells industry in the early years, but more of that later.
How exactly do solar PV cells work? Solar PV cells work by converting sunlight directly into electricity. Each PV cell is made of semiconductor materials, like silicon, which have special properties. In actual operation, the photons in the sunlight are absorbed by the semiconductor which causes the movement of the electrons, causing them to flow and create an electric current. This flow of electrons generates direct current (DC) electricity. The PV cells are connected together in a panel, and multiple panels are connected to form a solar array. The electricity produced by the solar array can then be used to power homes, buildings, or other electrical devices.
If the oil shocks of the late 1960s and early 1970s made the world aware of the potential of oil as a weapon, even before that, Exxon was the first oil company to understand the finite nature of this precious fossil source of energy and had begun to hedge its bet on other possible revenue streams. As the solar PV cells helped satellites get a reliable alternative to batteries, the idea caught up with an executive at Exxon as a solution to the batteries powering navigational aids on remote oil platforms. Typically, the batteries did not last long, were expensive to replace, and worse, were often discarded at sea, adding to the pollution. The work that Exxon started then to develop PV cells of its own, later led to the formation of its subsidiary, Solar Power Corporation, in 1973. Thanks to its huge revenues, Exxon could spend a large amount of resources for developing solar PV cells and panels, to help them spread beyond the use in satellites. Yet, in another twist of fate, the slow progress of solar power and its failure to capture popular imagination resulted in Exxon shutting down SPC in the mid-1980s, not willing to fund further research and wait for the dividends to follow. But the biggest blow the Exxon initiative struck for solar PV cells was to drive down the cost of solar power from $100 per watt to $20 a watt and paved the way for further research and reduction.
In the meanwhile, other initiatives followed. In 1977, the US Department of Energy launched the Solar Energy Research Institute (later renamed National Renewable Energy Laboratory) dedicated to research and development of solar energy. Governments and research institutions in other countries worldwide began also funding solar research and development. Efficiency improvements and cost reductions followed.
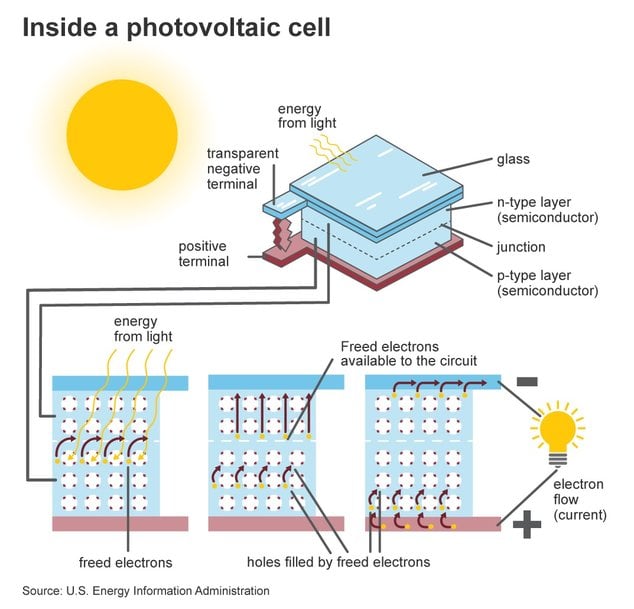
The schematic of a typical solar PV cell. Source: US Energy Information Department
Solar cell efficiency
According to the website of the US Government’s Office of Energy Efficiency & Renewable Energy, the amount of sunlight that strikes the earth's surface in an hour and a half is enough to handle the entire world's energy consumption for a full year. In reality though, it is not possible to convert this entire sunlight into electricity or useful thermal energy, and that is where the efficiency of the solar PV cell enters the equation, which is the amount of usable electrical power a solar cell produces from the sunlight shining on it. This efficiency is expressed as a percentage and represents the ratio of electrical power output from the solar cell to the solar power input from the sun. In simpler terms, it measures how effectively a solar cell can convert sunlight into electricity.
The efficiency of solar PV cells has been improving continuously with every passing year and development of new materials and processes. For example, the first practical solar cell developed by Bell Laboratories in 1954 had an efficiency of just around 6%. The progress in the initial years was very slow and in the decade that followed, efficiencies remained relatively low, typically below 10%. During the 1980s, efficiencies began to increase due to advancements in materials and manufacturing techniques and reached around 15%. The 1990s witnessed further improvements in materials and manufacturing processes that pushed efficiencies up to about 20%.
In the new millennium, efficiencies continued to climb steadily. By the end of the decade, efficiencies of around 25% were achieved in laboratory settings for some high-performance solar cells. Post-2010s, continued research and development led to incremental improvements, with efficiencies surpassing 30% in laboratory settings for certain types of solar cells. Technological advancements, including the development of new materials, improved cell designs, and enhanced manufacturing processes, have led to efficiencies exceeding 40% in laboratory settings for some cutting-edge solar cell technologies in the present time. But it may be noted that while laboratory efficiencies have continued to rise, the efficiencies of commercial solar panels available on the market today are typically only about 25% maximum.
There are several factors that impact the efficiency of solar PV cells and modules. These broadly include the intensity of the sunlight, cloud cover, the temperature of the solar panel, wind speed and altitude at which these panels are placed. Even the dust that settles on the solar panels impact their efficiency and it is recommended to clean the panels at least once in a year. Higher-efficiency solar cells can produce more electricity for a given amount of sunlight, making them more cost-effective and practical for various applications, including residential and commercial solar power systems. Improving solar cell efficiency is an ongoing goal in solar energy research and development.
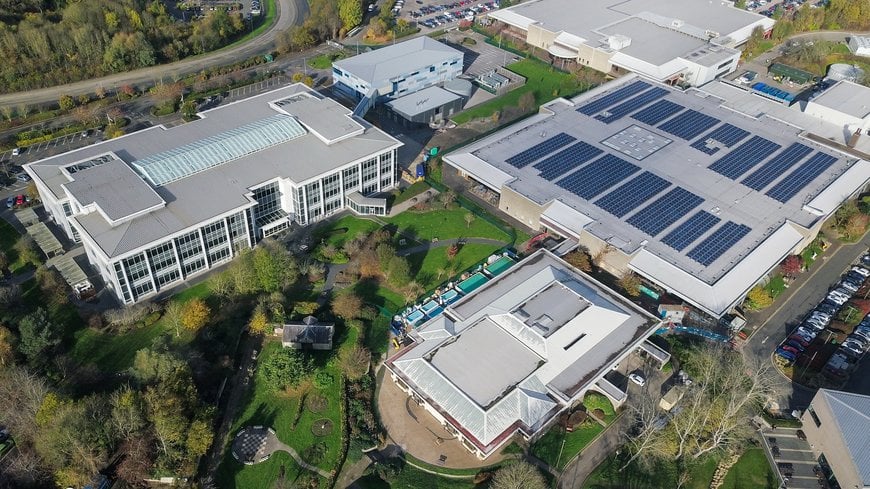
Aerial shot of industrial buildings in Bristol, UK. Photo by wirestock on Freepik
Types of solar PV cells
Solar photovoltaic (PV) cells come in several types, each with its own advantages and applications. Some of the common types of solar PV cells are described below:
Monocrystalline Silicon Solar Cells: These cells are made from a single crystal structure, typically sliced from cylindrical ingots. These were the first commercially available solar PV cells. They are highly efficient and space-saving, making them popular for residential and commercial installations. On the other hand, these cells are expensive and their manufacturing process is slow and labour-intensive.
Polycrystalline Silicon Solar Cells: These cells are made from silicon crystals that are melted together, then sawn and packaged similar to monocrystalline cells. As the name suggests, instead of a single uniform crystal structure, these cells contain many small grains of crystals. They are less expensive to produce than monocrystalline cells but are slightly less efficient. They have a bluish hue due to multiple crystals.
Thin-Film Solar Cells: Thin-film solar cells are made by depositing thin layers of photovoltaic material onto a substrate such as glass, metal, or plastic. Types of thin-film solar cells include:
- Amorphous Silicon (a-Si): Flexible and lightweight, but less efficient than crystalline silicon cells.
- Cadmium Telluride (CdTe): Offers higher efficiency compared to amorphous silicon and can be produced at lower cost.
- Copper Indium Gallium Selenide (CIGS): Known for its high-efficiency potential and flexibility.
Organic Photovoltaic Cells (OPVs): These cells use organic materials (carbon-based) to convert sunlight into electricity. They are lightweight, flexible, and can be produced using low-cost printing techniques. Theoretically, OPVs have the potential to provide electricity at a lower cost than first- and second-generation solar technologies. However, their efficiency is currently lower than traditional solar cells.
Perovskite Solar Cells: Perovskite solar cells are a relatively new type of solar cell that uses perovskite-structured materials. They offer high-efficiency potential and can be manufactured using low-cost methods like solution processing. However, they are still in the research and development stage and face challenges related to stability and scalability.
Each type of solar cell has its own set of advantages and disadvantages, and the choice of which to use depends on factors such as cost, efficiency, durability, and application requirements.
Innovations galore as solar power soars
The first solar PV cells ever were used on the US satellite Vanguard1 as noted at the beginning. It was a small panel measuring just 100 sq.cm with output of 0.1W, but could power the transmitter for close to 6 years. The solar PV cells have continued to evolve since then with gradual improvement in efficiency and dramatic reduction in cost. What was once a prohibitively expensive mode of electric power generation that was used exclusively for space and defence applications has today become one of the cheapest, and more importantly, eco-friendly way of power generation. Like Moore's Law regarding the number of transistors on an IC, there is an equivalent for solar power. Called Swanson's Law, after Richard Swanson, founder of American solar-cell manufacturer SunPower, this states that the cost of solar PV cells drops by 20% with each doubling of global manufacturing capacity.
What exactly are the innovations happening in solar PV cells? Here are a few key developments:
Perovskite Solar Cells: Invented in Japan, Perovskite solar cells (PSC) have garnered significant attention due to their high-efficiency potential and low production costs. PSCs are film-shaped solar cells made of a material whose crystal structure resembles that of a mineral called perovskite. Researchers are constantly improving the stability, scalability, and efficiency of perovskite materials, aiming to make them competitive with traditional silicon-based solar cells. The key advantage is these can be installed in places where conventional solar panels cannot, such as on the exterior walls of a building or on curved surfaces.
Tandem Solar Cells: Tandem or multijunction solar cells combine multiple semiconductor materials with varying bandgaps to capture a broader spectrum of sunlight. By stacking layers of different materials, tandem solar cells can achieve higher efficiencies than single-junction cells. Researchers are exploring various material combinations and fabrication techniques to optimise tandem solar cell performance.
Passivated Emitter and Rear Cell (PERC) Technology: PERC technology improves the efficiency of conventional silicon solar cells by adding a passivation layer to the rear surface of the cell, reducing electron recombination. PERC technology has become widespread in the solar industry and continues to see refinements for even higher efficiencies.
Bifacial Solar Panels: Bifacial solar panels can generate electricity from both the front and back sides, capturing sunlight reflected off the ground or nearby surfaces. Advanced bifacial panels incorporate optimised cell designs, transparent backsheets, and tracking systems to enhance energy generation.
Heterojunction Solar Cells: Heterojunction solar cells combine layers of different semiconductor materials to reduce energy losses at the cell interfaces. These cells exhibit high efficiency and improved performance in hot and low-light conditions. Manufacturers are scaling up production and refining manufacturing processes to make heterojunction cells more commercially viable.
Passive Cooling Technologies: Excessive heat can decrease solar cell efficiency. Researchers are developing passive cooling technologies such as selective emitters, thermal coatings, and novel cell designs to reduce operating temperatures and improve performance in high-temperature environments.
Advanced Light-Trapping Structures: Light-trapping structures, such as textured surfaces and nanostructures, increase the absorption of sunlight within the solar cell. Innovations in light-trapping techniques aim to enhance light absorption while minimising material usage and fabrication complexity.
Quantum Dot Solar Cells: Quantum dot solar cells utilize nanoscale semiconductor particles to tune the absorption spectrum of the cell and improve efficiency. Researchers are exploring novel quantum dot materials and device architectures to enhance performance and stability.
Flexible and Lightweight Solar Cells: Flexible and lightweight solar cells enable integration into a variety of applications, such as wearable devices, building-integrated photovoltaics (BIPV), and portable electronics. Innovations in flexible substrate materials and manufacturing processes are making these lightweight solar cells more durable and cost-effective.
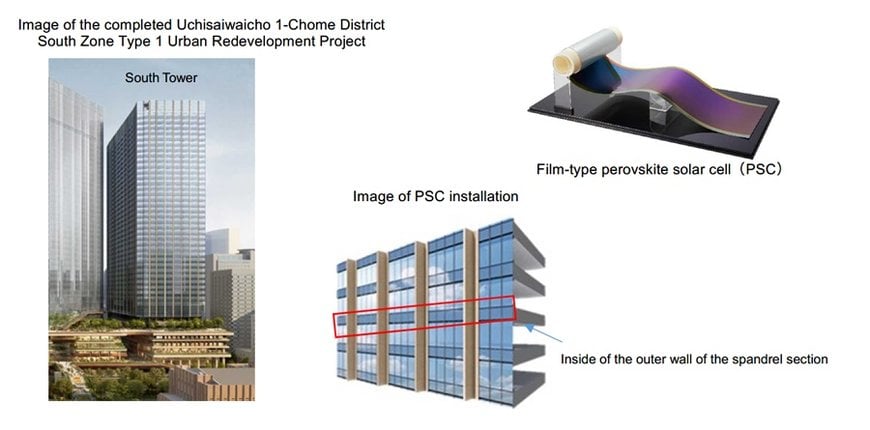
The proposed plan for the world's first mega solar power generation in a high-rise building using perovskite solar cells. Image source: Sekisui Chemical Co, Ltd, Japan.
These innovations, among others, contribute to the ongoing improvement of solar PV technology, driving increased efficiency, reduced costs, and broader adoption of solar energy worldwide.
Challenges in implementation
As seen in the preceding paragraphs, solar energy plays a crucial role in shaping a sustainable future. The reasons are not far to seek. Solar energy is not just abundant and renewable – it is a limitless source of energy that can be harnessed without degrading the earth or causing environmental damage. By shifting to solar power, humans can significantly reduce the carbon footprint and mitigate the impacts of global warming, which is the need of the hour. In a world ridden with conflicts, energy dependence is a major hindrance for development and progress. Solar energy allows communities and nations to become more energy independent by reducing reliance on imported fossil fuels. This can enhance energy security and stability, as well as reduce geopolitical tensions related to energy resources. With so many factors in favour of solar energy, what are the challenges that prevent wider adoption of this abundant resource for greater good?
There are in fact various challenges and hurdles in the path of wider adoption of solar power. These may be summarised in the following points:
High Initial Cost: Even as the payback period or return on investment (RoI) is getting compressed with falling prices and rising efficiencies of solar panels, the upfront cost of installing solar panels can still be significant. This is certainly a deterrent for many individuals and businesses from investing in solar energy despite its long-term benefits. Also not all governments have the resources to provide the much needed incentives and subsidies to promote solar to the extent they should.
Intermittent Power and Storage: Given the intermittent nature of solar energy, battery storage systems are essential to allow solar PV installations to provide a steady and dependable power supply, so that even when there is no sunlight, the energy stored during peak hours provides power when sunlight is not available.
Grid Integration: Integrating solar power into existing electricity grids can be complex, requiring upgrades to infrastructure and balancing supply and demand fluctuations. While most countries today have facilitated this through the distribution companies, problems remain as the variable and uncertain nature of solar PV systems pose certain technical and/or practical problems to the system operators.
Land Use and Infrastructure: Large-scale solar installations require significant land area, which can lead to conflicts with agriculture, conservation, and other land-use priorities. This is especially true for countries like India where land is in short supply given the huge population. Inadequate infrastructure like roads and basic electricity grid connectivity are other issues that can pose serious roadblocks.
Technological Limitations: Despite advancements, solar technology still faces efficiency limitations, such as converting sunlight into electricity at a relatively low efficiency rate compared to other energy sources.
Weather Dependence: Solar power generation can be affected by weather conditions such as clouds, rain, and atmospheric pollution, leading to fluctuations in output.
Supply Chain Issues: The availability of raw materials, such as rare earth elements used in solar panels, can be affected by geopolitical factors and supply chain disruptions.
Environmental Concerns: As the industry grows, there are concerns related to PV waste and recycling. According to the International Energy Agency (IEA), if panels are systematically collected at the end of their lifetime, supplies from recycling them could meet over 20% of the solar PV industry’s demand for aluminium, copper, glass, silicon and almost 70% for silver between 2040 and 2050. But at present, existing PV recycling processes struggle to generate enough revenue from the recovered materials to cover the cost of the recycling process.
Addressing these challenges requires continued technological innovation, supportive policies, public education, and investment in infrastructure to unlock the full potential of solar power as a sustainable energy source.
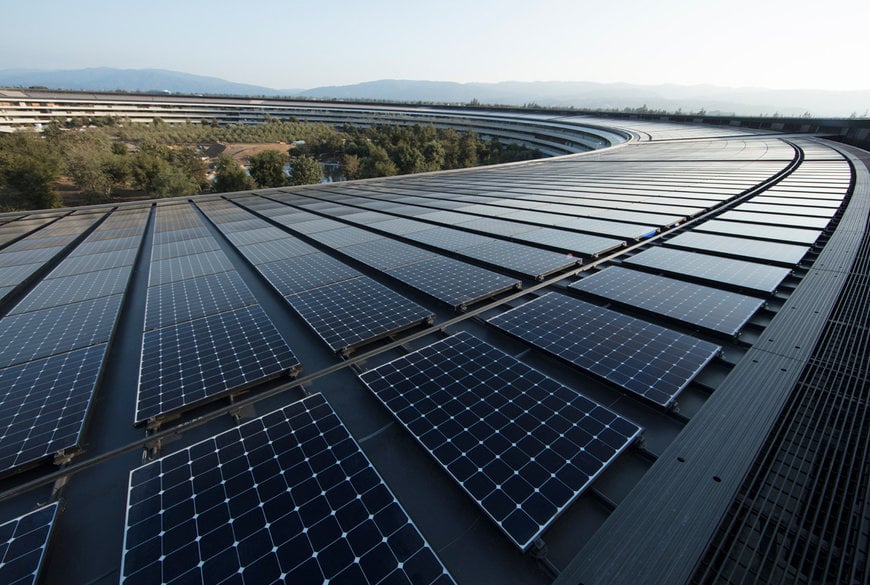
Apple’s new headquarters in Cupertino is powered by 100 percent renewable energy, in part from a 17-megawatt onsite rooftop solar installation. Image source: Apple
Conclusion
The latest statistics from the IEA state the world added 50% more renewable capacity in 2023 than in 2022 and the next 5 years will see the fastest growth yet; but lack of financing for emerging and developing economies is a key issue that needs serious attention from governments and policymakers. The report further notes that the world’s capacity to generate renewable electricity is expanding faster than at any time in the last three decades, giving it a real chance of achieving the goal of tripling global capacity by 2030 that governments set at the COP28 climate change conference in late 2023 in UAE.
The world’s first solar park was built near California in the US in 1982, and had a capacity of 1 MW. Today, most solar parks have an installed capacity of 550 MW of electricity. The largest solar park in the world is the Bhadla Solar Park in Rajasthan, India with a total installed capacity of 2,245 MW. The world is scaling up solar power generation, and that augurs well for Planet Earth!
References
1. https://en.wikipedia.org/wiki/Solar_cell
2. https://www.npr.org/2019/09/30/763844598/how-big-oil-of-the-past-helped-launch-the-solar-industry-of-today
3. https://sj.jst.go.jp/stories/2023/s0929-01j.html
4. https://www.nrel.gov/news/program/2024/nrel-researchers-outline-path-forward-for-tandem-solar-cells.html
5. https://www.iea.org/news/massive-expansion-of-renewable-power-opens-door-to-achieving-global-tripling-goal-set-at-cop28
Perovskite Solar Cells: Perovskite solar cells are a relatively new type of solar cell that uses perovskite-structured materials. They offer high-efficiency potential and can be manufactured using low-cost methods like solution processing. However, they are still in the research and development stage and face challenges related to stability and scalability.
Each type of solar cell has its own set of advantages and disadvantages, and the choice of which to use depends on factors such as cost, efficiency, durability, and application requirements.
Innovations galore as solar power soars
The first solar PV cells ever were used on the US satellite Vanguard1 as noted at the beginning. It was a small panel measuring just 100 sq.cm with output of 0.1W, but could power the transmitter for close to 6 years. The solar PV cells have continued to evolve since then with gradual improvement in efficiency and dramatic reduction in cost. What was once a prohibitively expensive mode of electric power generation that was used exclusively for space and defence applications has today become one of the cheapest, and more importantly, eco-friendly way of power generation. Like Moore's Law regarding the number of transistors on an IC, there is an equivalent for solar power. Called Swanson's Law, after Richard Swanson, founder of American solar-cell manufacturer SunPower, this states that the cost of solar PV cells drops by 20% with each doubling of global manufacturing capacity.
What exactly are the innovations happening in solar PV cells? Here are a few key developments:
Perovskite Solar Cells: Invented in Japan, Perovskite solar cells (PSC) have garnered significant attention due to their high-efficiency potential and low production costs. PSCs are film-shaped solar cells made of a material whose crystal structure resembles that of a mineral called perovskite. Researchers are constantly improving the stability, scalability, and efficiency of perovskite materials, aiming to make them competitive with traditional silicon-based solar cells. The key advantage is these can be installed in places where conventional solar panels cannot, such as on the exterior walls of a building or on curved surfaces.
Tandem Solar Cells: Tandem or multijunction solar cells combine multiple semiconductor materials with varying bandgaps to capture a broader spectrum of sunlight. By stacking layers of different materials, tandem solar cells can achieve higher efficiencies than single-junction cells. Researchers are exploring various material combinations and fabrication techniques to optimise tandem solar cell performance.
Passivated Emitter and Rear Cell (PERC) Technology: PERC technology improves the efficiency of conventional silicon solar cells by adding a passivation layer to the rear surface of the cell, reducing electron recombination. PERC technology has become widespread in the solar industry and continues to see refinements for even higher efficiencies.
Bifacial Solar Panels: Bifacial solar panels can generate electricity from both the front and back sides, capturing sunlight reflected off the ground or nearby surfaces. Advanced bifacial panels incorporate optimised cell designs, transparent backsheets, and tracking systems to enhance energy generation.
Heterojunction Solar Cells: Heterojunction solar cells combine layers of different semiconductor materials to reduce energy losses at the cell interfaces. These cells exhibit high efficiency and improved performance in hot and low-light conditions. Manufacturers are scaling up production and refining manufacturing processes to make heterojunction cells more commercially viable.
Passive Cooling Technologies: Excessive heat can decrease solar cell efficiency. Researchers are developing passive cooling technologies such as selective emitters, thermal coatings, and novel cell designs to reduce operating temperatures and improve performance in high-temperature environments.
Advanced Light-Trapping Structures: Light-trapping structures, such as textured surfaces and nanostructures, increase the absorption of sunlight within the solar cell. Innovations in light-trapping techniques aim to enhance light absorption while minimising material usage and fabrication complexity.
Quantum Dot Solar Cells: Quantum dot solar cells utilize nanoscale semiconductor particles to tune the absorption spectrum of the cell and improve efficiency. Researchers are exploring novel quantum dot materials and device architectures to enhance performance and stability.
Flexible and Lightweight Solar Cells: Flexible and lightweight solar cells enable integration into a variety of applications, such as wearable devices, building-integrated photovoltaics (BIPV), and portable electronics. Innovations in flexible substrate materials and manufacturing processes are making these lightweight solar cells more durable and cost-effective.
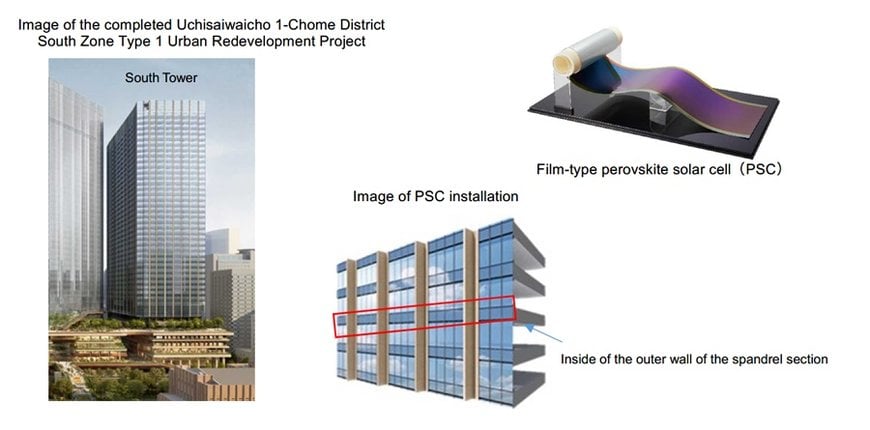
The proposed plan for the world's first mega solar power generation in a high-rise building using perovskite solar cells. Image source: Sekisui Chemical Co, Ltd, Japan.
These innovations, among others, contribute to the ongoing improvement of solar PV technology, driving increased efficiency, reduced costs, and broader adoption of solar energy worldwide.
Challenges in implementation
As seen in the preceding paragraphs, solar energy plays a crucial role in shaping a sustainable future. The reasons are not far to seek. Solar energy is not just abundant and renewable – it is a limitless source of energy that can be harnessed without degrading the earth or causing environmental damage. By shifting to solar power, humans can significantly reduce the carbon footprint and mitigate the impacts of global warming, which is the need of the hour. In a world ridden with conflicts, energy dependence is a major hindrance for development and progress. Solar energy allows communities and nations to become more energy independent by reducing reliance on imported fossil fuels. This can enhance energy security and stability, as well as reduce geopolitical tensions related to energy resources. With so many factors in favour of solar energy, what are the challenges that prevent wider adoption of this abundant resource for greater good?
There are in fact various challenges and hurdles in the path of wider adoption of solar power. These may be summarised in the following points:
High Initial Cost: Even as the payback period or return on investment (RoI) is getting compressed with falling prices and rising efficiencies of solar panels, the upfront cost of installing solar panels can still be significant. This is certainly a deterrent for many individuals and businesses from investing in solar energy despite its long-term benefits. Also not all governments have the resources to provide the much needed incentives and subsidies to promote solar to the extent they should.
Intermittent Power and Storage: Given the intermittent nature of solar energy, battery storage systems are essential to allow solar PV installations to provide a steady and dependable power supply, so that even when there is no sunlight, the energy stored during peak hours provides power when sunlight is not available.
Grid Integration: Integrating solar power into existing electricity grids can be complex, requiring upgrades to infrastructure and balancing supply and demand fluctuations. While most countries today have facilitated this through the distribution companies, problems remain as the variable and uncertain nature of solar PV systems pose certain technical and/or practical problems to the system operators.
Land Use and Infrastructure: Large-scale solar installations require significant land area, which can lead to conflicts with agriculture, conservation, and other land-use priorities. This is especially true for countries like India where land is in short supply given the huge population. Inadequate infrastructure like roads and basic electricity grid connectivity are other issues that can pose serious roadblocks.
Technological Limitations: Despite advancements, solar technology still faces efficiency limitations, such as converting sunlight into electricity at a relatively low efficiency rate compared to other energy sources.
Weather Dependence: Solar power generation can be affected by weather conditions such as clouds, rain, and atmospheric pollution, leading to fluctuations in output.
Supply Chain Issues: The availability of raw materials, such as rare earth elements used in solar panels, can be affected by geopolitical factors and supply chain disruptions.
Environmental Concerns: As the industry grows, there are concerns related to PV waste and recycling. According to the International Energy Agency (IEA), if panels are systematically collected at the end of their lifetime, supplies from recycling them could meet over 20% of the solar PV industry’s demand for aluminium, copper, glass, silicon and almost 70% for silver between 2040 and 2050. But at present, existing PV recycling processes struggle to generate enough revenue from the recovered materials to cover the cost of the recycling process.
Addressing these challenges requires continued technological innovation, supportive policies, public education, and investment in infrastructure to unlock the full potential of solar power as a sustainable energy source.
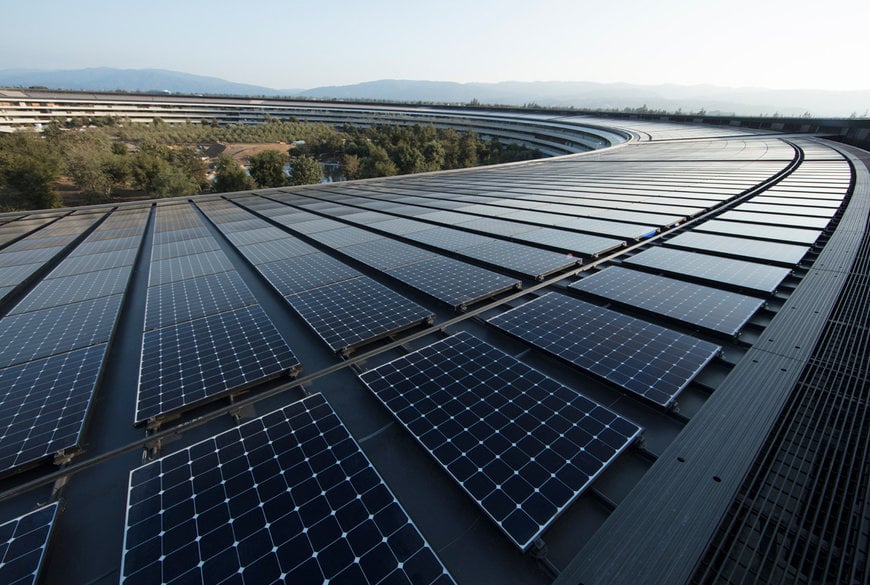
Apple’s new headquarters in Cupertino is powered by 100 percent renewable energy, in part from a 17-megawatt onsite rooftop solar installation. Image source: Apple
Conclusion
The latest statistics from the IEA state the world added 50% more renewable capacity in 2023 than in 2022 and the next 5 years will see the fastest growth yet; but lack of financing for emerging and developing economies is a key issue that needs serious attention from governments and policymakers. The report further notes that the world’s capacity to generate renewable electricity is expanding faster than at any time in the last three decades, giving it a real chance of achieving the goal of tripling global capacity by 2030 that governments set at the COP28 climate change conference in late 2023 in UAE.
The world’s first solar park was built near California in the US in 1982, and had a capacity of 1 MW. Today, most solar parks have an installed capacity of 550 MW of electricity. The largest solar park in the world is the Bhadla Solar Park in Rajasthan, India with a total installed capacity of 2,245 MW. The world is scaling up solar power generation, and that augurs well for Planet Earth!
References
1. https://en.wikipedia.org/wiki/Solar_cell
2. https://www.npr.org/2019/09/30/763844598/how-big-oil-of-the-past-helped-launch-the-solar-industry-of-today
3. https://sj.jst.go.jp/stories/2023/s0929-01j.html
4. https://www.nrel.gov/news/program/2024/nrel-researchers-outline-path-forward-for-tandem-solar-cells.html
5. https://www.iea.org/news/massive-expansion-of-renewable-power-opens-door-to-achieving-global-tripling-goal-set-at-cop28